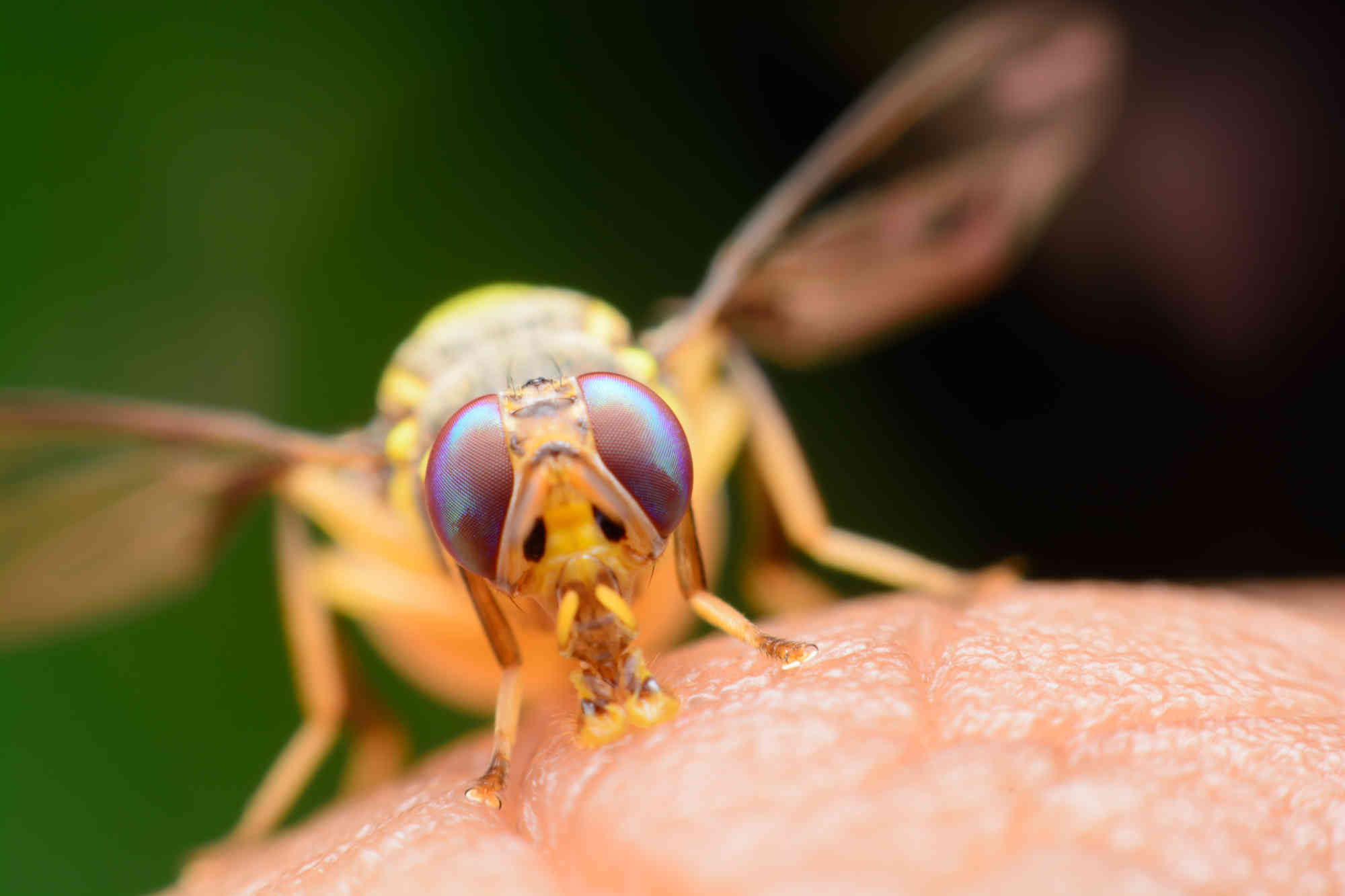
How Does Nature Do It?
Steve Jones, April 18, 2023
Just how hard is it to create a brain? Nature provides us with virtually uncountable examples of working brain hardware that enable animals to live productive lives.
Despite the ubiquity and variety of nature's invention, even defining the most basic functionality or describing how brains work at any level has eluded humans interested in them for centuries. Initially in fact, it was thought that the brain was actually an organ that warmed the blood. It was centuries later that we came to realize that brains were actually composed of neurons, and single neurons were isolated and their electrochemical properties studied in the laboratory. In the mean time, various studies of accidental and surgical lesions of the brain yielded broad-strokes information, such as particular areas of the human brain generally being responsible for generally-defined tasks. As the human brain (and countless brains of lab rats, cats, and monkeys) became more mapped, general hypotheses were formed about how these areas might be connected and form a single working organ. Through scientific study (especially more recently, with the use of non-invasive fMRI real-time imaging of general brain usage of oxygen as a metric of which areas might be more active) we have still failed to really understand why we have brains and how they came about in the first place.
Life Version 1: Bacteria
At the dawn of life, there was chemistry, and plenty of stochastic interactions at various temperatures and pressures to create identifiable systems that were able to clone themselves. Some of these chemical patterns went on to become single cell bacteria that moved with external appendages attached to the outer cell wall, which moved when the cell wall was could detect a greater concentration of nutrient chemicals at one end of the animal than the other, allowing the cell to eventually move in the direction of better nutrition. It became a winning strategy for organisms to be longer or more shapely, so as to increase sensitivity, and to have better locomotion mechanisms. Nothing so far required a brain, so there was none; it was simply hard-wired, and evolution experimented with plenty of variations. Simply through exhaustive stochastic search, organisms evolved to become multi-cellular, and this invention led to the encoding of the animal's body structure into RNA, as plants took over the world.
Life Version 2: Plants
Plants are sessile; they don't move on their own. Brains still didn't exist, because there was no need for them to do so from the beginning. Using nutrition that simply passes nearby can be an effective strategy for the lucky individuals that are situated in the right places. Communication inside plants and between individuals was accomplished with chemical gradients, on a timescale 104 to 108 times slower than our sensory world does. Plants even evolved to coordinate with fungus, another kingdome of sessile organisms, offloading the tasks of networking the underground communication between plants, as well as emergency nutrition sharing. One of the most salient features of plant architecture is that it is simple-- they have not in general evolved new capabilities, but rather have evolved strategies for making their simplicity work even in harsh environments.
Despite not having brains, plants do exhibit behavior. They sense toxins, nutrition, and communication from other plants as chemistry in the environment, and they can take action, including increasing or limiting growth of foliage or protective films on their bodies, as well as extending their roots to make contact with nutrition, water, and fungus to become better connected.
A behavior we can appreciate as mobile animals is plants' ability to respond to light by bending their bodies toward the light source, to increase the possibility of radiation absorption. We have all seen time-lapse movies of plants following the sun, but what can be shown in the laboratory is fascinating. If a single blade of common lawn grass is grown in a little Dixie cup, it can follow the light of a candle 10' away, even in an otherwise totally dark room. Try this: Place the grass at one end of a completely dark room, place a small candle at the other end (even 20-30 feet away), and leave them overnight. In the morning, the blade of grass will have bent toward the candle. Is there a specific part of the grass that senses the light?
To answer this question, plant six separate blades of grass in separate pots. Cover one blade with a thin clear plastic, another with an opaque clear plastic. Then do the same for two more sets, only covering the top of the blade or the bottom of the blade, respectively. What we find is pretty interesting: The blades that allow light to pass to their leaf tips still bend, but the others do not. In other words, blades of grass "see" the light with the tip of their body's leaf. And, the bending happens elsewhere in the stalk of the blade, communicated with chemistry by the leaf-tip about which direction the candle is. Grass blades then, sense the environment and take physical action based on that information to benefit the organism, all without muscles or sophisticated eyeballs with lenses and retinas.
Of course, we don't often think of single blades of grass on their own. We do encounter large planar arrays of grass blades packed close together-- we call them lawns. And of course it is unlikely that blades of grass give up their "seeing" behavior in large groups. In fact, you can see this behavior in lawns in time-lapse photography in your very own yard. What is interesting to think about in this scenario is that the population of grass blades in a lawn has individuals that each see the world from a slightly different vantage point. They are, in a sense, very large retinal arrays. And in summer, perhaps every three weeks, we mow off all the eyes, and blind them. They then grow new eyes and use them to see the world until the next mowing. We have to wonder, just how much do we really appreciate about plants, if we are only coming to realize that it is conceivable that large populations of plants might function as single organisms, yet on a timescale that is so slow, that humans just don't notice their behavior and are therefore ignorant of it?
Life Version 3: Animals
While plants operated on a slow timescale and took the streamlined simple architectural approach that relied on group populations and fungus to take care of the complexities of surviving in the world, nature was quite busy with trying another strategy as well-- animals, the fast movers of the world. Unlike their sessant cousins, animals evolved with very creative body plans and behavioral strategies to work in the fast lane. The idea was to move toward the nutrients, away from the predators, and find the best qualified mates. In order to do these things, locomotion was necessary, and that involved elaborating on bacteria's cillia appendages. Cellular differentiation became rampant and soon there were cells for building muscles, different organs, and even nerve cells, which could transmit information much faster than the chemical pumps in plant bodies.
Fast internal signalling made it useful for sensory organs to sense on a much faster timescale, so that instead of general light level and direction, detection of movement was possible, as was a sense of smell, electric fields on fishes' lateral line, and touch-- especially to be able to respond when the body being attacked by another animal. With information transfer rates on the order of milliseconds instead of hours, the behavioral timescales we recognize as relevant as humans, became important. While initially reflex-arc wiring could enable primitive muscles to move an appendage or spray a toxin in defense, increasingly sophisticated behaviors would be significantly rewarded. This required a new structure-- a neural nucleon, or network of neurons. It is estimated that these first brains appeared some 500m years ago.
Today we can study contemporary primitive animals with these rudiments of brains-- the nematode (flatworm) we call C. Elegans. This animal has a fixed-wired 302-neuron nucleon that completely defines its behavior (actually, there are two variants of this animal, a male and a hermaphrodite, so there are two brain versions, one with a few more neurons to handle the female body parts in the hermaphrodite). These animals can seek out nutrition, flee predators, and even mate with other worms, on a timescale plants cannot achieve with their chemistry-based hardware.
What is significant about these early organisms with fixed-wired brains is that they are the perfect laboratory for nature's ongoing experiments. Adding just a few more neurons could significantly improve the behavioral complexity of an organism and enable them to have a clear evolutionary advantage. And the timescale at which these experiments could take place had changed by several orders of magnitude. More and more complex brains were created until finally their complexity outstripped the ability of the genome to encode it. A new strategy was developed-- create complexity as space allows in the general body plan, and let the network self-organize with plasticity in the connections between the nodes in the network. Further experiments included the development of different types of neurons, different types of neurotransmitters and signalling mechanisms, and different kinds of receptors used by the neurons to detect and process signals.
This phase exhibited a huge range of successful prototype brains that nature used to support a wide range of body plans for its zoo of creatures. Brain plans were high-level, not down to the individual neuron, but rather describing the raw materials to be used by individual nuclei, and how the nuclei with matching signalling systems could connect. A trunk-level schematic with brain "areas" was therefore prescribed by a set of nuclei and signal types. This is the brain of insects.
A lot can be learned by the study of insect brains, because insects are highly capable animals that lead productive lives with behavior on a timescale that we can recognize and study. Yet, these brains do not employ nearly as many neurons as one might think would be needed to cause behavior to arise-- a honeybee, as well as a cockroach, both have brains with approximately one million (106) neurons. These animals are social, work together, defend themselves and their society, can hunt, and communicate with one another. Much smaller still is Drosophila Melanogaster, a common fruit fly, clocking in at 200,000 (2x105) neurons. These flies are not as social, but can hunt for food, evade predators, and reproduce. They lead a full, albeit short, productive life with plenty of movement in the world, all with only 200,000 neurons generating their behavior. And it is interesting that most of the neurons in this animal's brain are devoted to processing visual information from their sophisticated eyes. If only 20% of this animal's brain is used to generate real behavior, then that's 0.20 x 200,000 = 40,000 neurons that produce behavior allowing the organism to successfully navigate the world.
Life Version 3.1: Early Brains
Nature's next significant optimization was myelinated axons that allowed signals to transmit 10x faster than unmyelinated ones in existing brain models. This enabled signal sources and muscles to be placed further away from the brain itself, so animals could be much larger. The first set of animals (dinosaurs) used a simple brain architecture that must have evolved from insect brains; we now call this the reptilian brain.
Nature used the reptilian brain architecture as a platform on which new designs could be based. One of these, the limbic system, was an add-on to the reptilian brain that could provide emotionally-based behavioral responses, optimizing performance of the animal with this special processing unit.
Another new invention was the cerebellum, a dense cortex at the back of most brains that provides precision-- it is nature's coprocessor. Movements in animals with damaged cerebellumns are clumbsy, but still possible. This is the system that makes "muscle memory" work-- how we learn by practicing movements as musicians and atheletes. The cerebellum is also thought to process other input, such as pain. In humans, most of the neurons are located not in the cerebral cortex, but in the cerebellum; of the 87 billion in total, only 16 billion are in the cerebrum.
Life Version 3.2: Cerebral Cortex
Previously, the reptilian brain had been organized as a set of nuclei with plastic but stochastically-wired neural networks, much as insect brains are. Roughly 200m years ago, nature's experiments with neurogenesis must have produced a successful nucleon that had a more regular architecture-- a roughly three-layer cerebral cortex with regular cortical columns, with each of the layers containing a different combination of neuron types. This allowed for a repeating circuit that turned out to be effective as a computing platform for more general-purpose use, called a pallium in birds and fish.
In fact, this computing platform was a sheet of neural network fabric, and better results were achieved with larger arrays of the fabric, so that in order to grow larger within a given cranium size, the sheet had to fold in upon itself as it grew. The computational capacity of such a network was larger than the network of nuclei containing more randomized plastic architectures. The plasticity remained, and as the nucleon method was working, the new system was wrapped around the old system, rather than used as a replacement. Today's birds exhibit this architecture. They exhibit very sophisticated behavior, including significantly increased language and problem-solving abilities. Some birds can use tools to solve problems.
Life Version 3.3: Mammals
Along with a significant update associated with temperature regulation, a new group of animals called mammals developed an even better version of the cortex called the neocortex, a six-layer version (in most areas, while in some areas, it is still three deep). This new architecture resulted in a significantly enhanced set of behaviors, including the ability to make and use tools and create artificial languages, develop mathematics, record history, and perhaps most importantly-- to cook.
Cooking turned out to be a really important skill for humans, for with it, raw foods with limited caloric content could be cooked to increase the caloric intake and preserve food. This meant that the brain was taking on a task that was limiting the size of brains all along-- since a human brain takes approximately 25% of a human's energy intake to run.
Take Aways
What can we learn from this tour of brain evolution? First, there have been many different brain architectures explored by nature-- there have been billions of species with brains of various sorts. And of each of these species, there have been countless (billions to quadrillions) of individual animals with different wirings that still express the brain plan for their species. Impressively, they all work. Of course, results vary. Some individuals are more capable than others for certain behaviors, which ultimately leads to evolutionary selection. Also, there must be some brain wire-ups that worked so poorly that the individuals did not survive. So while we don't know what percentage of individuals for all species fail or succeed due to wire-up differences, we do know that for humans, the success rate is actually pretty high, without regard to whether the failures are due to brain wire-up issues or other abnormalities. In summary, nature has shown us that there are many ways to build a brain. In fact, it's hard to build one that doesn't work. This is good motivation for NeuroSynthetica's work-- with so many natural brains having success, surely we can find one that works. It's unlikely to be better at some behaviors than natural brains, with the number of experiments that nature has run, but certainly there must exist a good starting point.
Second, success doesn't mean creating a brain that is 87 billion neurons large. Even small brains, like that of Drosophila Melanogaster, can exhibit remarkable behaviors that are clearly recognizable as goal-directed, coordinated movements. Robots with even this level of intelligence will improve tremendously, having autonomous gaits, goal-seeking, and learning how the world around them works without it being spelled out for them with a fixed control regimen coded in Python or C.
We can also learn a lot by observing the road that was taken to get to that 87 billion neuron brain. We can start with imagining what evolutionary forces were at work in the early stages, and how those forces must have guided nature's stochastic evolutionary experiments. Starting with the simpler reptilian or even insect brains has the potential to produce remarkable results.
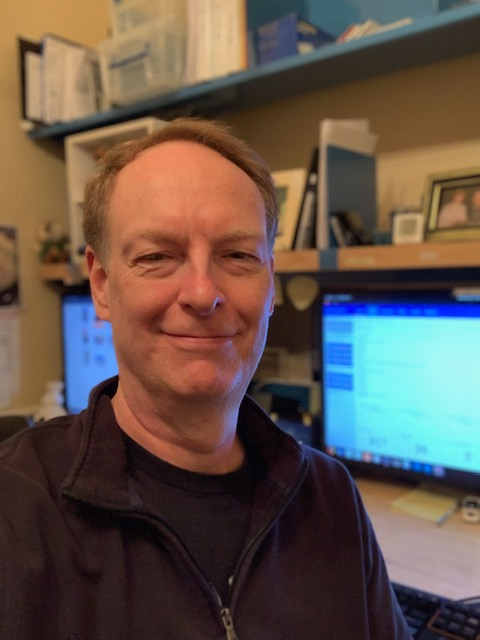
Steve Jones
Founder & CTO
Explorations into synthetic sentience and building the robotics used to demonstrate it.